The word “bionic” conjures up sci-fi visions of humans grown to superhuman levels. It is true that engineering advances such as better motors and batteries, along with modern computing, mean that the necessary mechanical and electronic systems are no longer a barrier to advanced prostheses. But the field has struggled to integrate these powerful machines with the human body.
This is beginning to change. A recent trial tested a new integration technique, which involves surgically reconstructing muscle pairs that give recipients a sense of position and movement of a bionic limb. Signals from those muscles control the robotic joints, so the prosthesis is completely under the control of the user’s brain. The system enabled people with below-the-knee amputations to walk more naturally and better navigate slopes, stairs and obstacles, researchers reported in July. Nature Medicine.
Engineers have typically seen biology as a fixed constraint to design, says bioengineer Tyler Clites, who helped develop the technique several years ago while at MIT. “But if we look at the body as part of the system to be designed, in parallel with the car, the two will be able to interact better.”
This look is fueling a wave of techniques that reengineer the body to better integrate with the car. Clites, now at UCLA, calls such techniques “anatomical,” to distinguish them from traditional bionics. “The issue we were dealing with was not an engineering problem,” he says. “The way the body was manipulated during the amputation was not leaving it in a position to be able to control the limbs we were creating.”
In an anatomical approach, bones are used to provide stable anchors; nerves are rerouted to create control signals for robotic limbs or transmit sensory feedback; muscles are chosen as biological amplifiers or grafted into place to provide more signal sources. All of these techniques improve the connection and communication between a robotic limb and the human nervous system, increasing what bionic prostheses can do (SN: 2/9/24).
Anatomically based devices have been slow to get out of the lab and into the commercial and clinical world. But some say the field is moving closer to that sci-fi vision of seamlessly integrated, brain-controlled bionic limbs — especially as more advances are on the horizon.
Here’s a closer look at how researchers aim to marry body and machine.
Muscle reconstruction
Proprioception—the body’s awareness of itself in space—is a tricky sense to regain, but it’s important for movement, especially walking (SN: 9/9/19). Muscles send signals to our brain about where our body is, how it moves and what forces it encounters. These signals are mainly generated by paired muscles called agonist-antagonist pairs, where one contracts while the other stretches.
In a traditional amputation, this important reaction is discarded. But the technique reported in the July study, known as an agonist-antagonist myoneural interface, or AMI, surgically reconstructs these push-pull pairs and uses the signals they generate to control prosthetic joints. The procedure allows the recipient to “feel” their prosthetic limb.
“When the prosthesis moves, the person actually feels that movement as a natural proprioceptive sensation,” says MIT bioscientist Hugh Herr, who developed the technique along with Clites and team surgeon Matthew Carty.
The latest study was part of a clinical trial that Herr and colleagues are conducting, which tested the technique in 14 people with below-the-knee amputations. Seven participants had undergone the AMI procedure, while the others had standard amputations. Recipients of the AMI-based system increased their walking speed by about 40 percent from 1.26 meters per second to 1.78 meters per second, the researchers found, a rate comparable to that of people without amputation.
Bone expansion
The most common complaints from denture wearers include pain and discomfort. A major source of concern is the attachment point.
“Many of the problems with using prostheses are related to the socket,” says bioengineer Cindy Chestek of the University of Michigan in Ann Arbor. Sour meat is not suitable for transferring loads to the part of the body built for that work – the bones. The resulting strain can cause tissue damage and, invariably, discomfort, sometimes causing users to abandon their device.
A technique called osseointegration takes advantage of the fact that certain metals bond with bone. A titanium bolt inserted into the skeleton anchors the prosthesis in place, providing greater strength, stability and comfort. “There’s a reason we have skeletons,” Chestek says.
The procedure was first performed in 1990, but did not become widely accepted and clinically available until the last decade. An implant system, called OPRA, received approval from the US Food and Drug Administration in 2020. The main drawback is that the titanium bolt must pass through the skin, creating a permanent hole that carries risks of infection. “Besides the risk of infection, osseointegration is better in every way,” says Chestek.
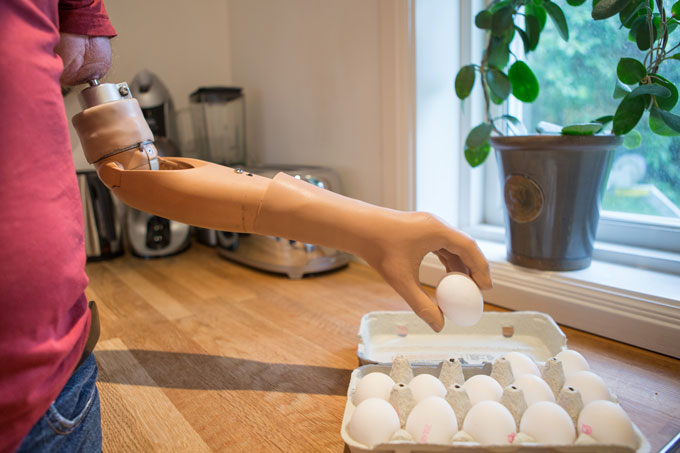
Redirection of nerves
Bionicists have long sought to tap into the body’s nerves to create prostheses that communicate with the brain. But early efforts were disappointing, largely because the signals carried by nerves are so weak.
“People have tried for decades to get meaningful signals from [putting] a wire inside a nerve,” says Chestek. “To date, it’s almost impossible outside of a controlled laboratory environment.”
Instead, modern bionic prostheses communicate primarily with muscles. When activated by a nerve, the muscles emit much larger electrical signals, which can be picked up by electrodes on the skin, which then control the prosthetic limb.
But the nerves that previously operated parts of a missing limb—and could similarly efficiently operate the artificial limb—usually don’t terminate in muscles. They don’t go anywhere, which creates neuromas, bulbs at nerve endings, whose electrical “spark” causes pain.
A procedure called targeted muscle reinnervation, or TMR, solves this problem. A surgeon removes the muscles from their native nerves and reroutes the severed nerves to this newly cleared ground. Rerouted nerves grow into muscles over time, which act as amplifiers, creating sources of the necessary control signals. “You turn a neural recording problem into a muscle recording problem,” Chestek says. “Registering muscles is easy.” The procedure also treats neuroma pain—a purpose for which it is often performed.
One drawback is that TMR cannibalizes existing muscles, limiting the number of signals that can be generated. “You run out of real estate very quickly,” says Chestek. This is especially important for above-the-knee or elbow amputations, where there is less muscle left and more prosthetic joints to control.
A new technique, known as a regenerative peripheral nerve interface, or RPNI, surgically inserts tiny muscle grafts taken from elsewhere and reroutes nerves to them instead. Surgeons can then spread these nerve bundles into their constituent fibers to take advantage of the newly grafted targets, allowing researchers to create as many signals as they need, Chestek says.
However, the small size of muscle grafts makes it difficult to obtain signals from them using surface electrodes. “You can’t record [electrical signals] from a three-centimeter piece of muscle through the skin very easily,” Chestek says. “You have to use implanted electrodes.” That’s more invasive, and implants face regulatory hurdles, but implanted electrodes produce higher-quality signals. They just must be accessed somehow, as passing wires through the skin is not feasible outside of laboratory studies.
Some researchers are working on wireless systems, but another solution is to combine RPNIs with osseointegration. In this setup, the wires between the implanted electrodes and the prosthesis simply pass through the titanium bolt. A study published last year described a bionic arm above the elbow using this approach that enabled the recipient to control each finger of his robotic hand.
Reconstruction of bodies
In his anatomy lab at UCLA, Clites says, “I have nine or 10 active collaborations with surgeons on different projects.” Here, he and his team use cadavers to test ideas and gather data. “We’ll mount the cadaver limbs on a manipulator arm and evaluate the systems we’re developing to make sure they work as intended,” says Clites. “It’s the backbone of what we do.”
One of the projects under development is a new bonding method that avoids the permanent hole that comes with osseointegration. Instead of a titanium bolt, there is a piece of steel in the limb and an electromagnet in the socket of the prosthesis. “That magnet holds [the socket] on the limb,” Clites says, “and then you can control how much pulling force there is by varying the current through that electromagnet. The plug must not carry loads; the magnetic force does the work, changing from moment to moment according to requirements, such as walking versus standing.
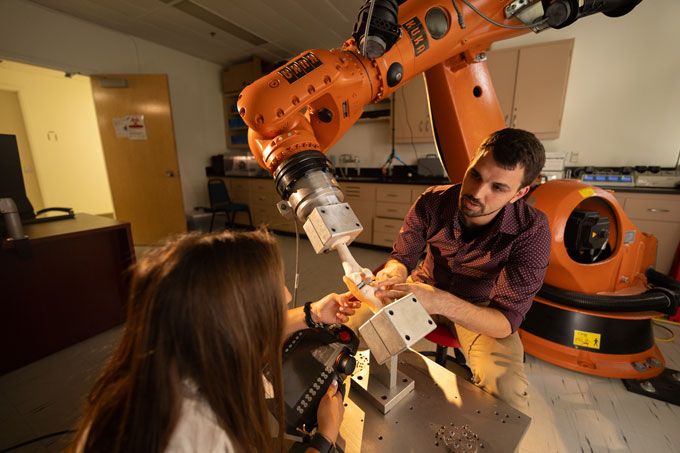
At MIT, Herr is also working on a new breakthrough. The latest trial of AMI-based bionic legs used electrodes on the skin to conduct signals from muscles to prosthetic joints. But surface electrodes have drawbacks, such as movement causing signal distortions. The new technique – called magnetomicrometry – involves placing magnetic spheres inside muscles and monitoring their movement with magnetometers. “With these magnets,” says Herr, “we can measure what we’re interested in and use that to directly control the bionic prosthesis.” A commercial product will be around for about five years, he says.
For God, such advances are personal. Both his legs were amputated below the knee after a mountain climbing accident 42 years ago. He is thinking of improving AMI-based bionic leg prostheses in the coming years. Once these techniques are perfected, he envisions a step forward. “When you marry surgical techniques like AMI and RPNI with something like magnetomicrometry, we believe it’s game-changing,” says Herr. “We believe there will be the Hollywood version of brain-controlled robotic limbs.”
An added benefit of restoring proprioception, in addition to other types of sensory feedback such as touch, is that it makes recipients feel more like a prosthesis is a part of them (SN: 22.4.21). “The goal in the field is when we do robotic reconstruction, the person says, ‘Oh my God, you gave me my body back,'” says Herr. “Instead of a robotic tool, we give them an entire hind limb. The field is very close to that objective.”
#Braincontrolled #bionic #limbs #closer #reality
Image Source : www.sciencenews.org